Conductivity refers to a material’s ability to allow the flow of electric charge. Good conductivity indicates electrons can move freely through the substance when voltage gets applied. Metals tend to demonstrate high electrical conductivity capacities.
The question around its electrical traits emerge among builders, engineers and scientists, Will stainless steel conduct electricity? Evaluating its conductivity properties requires analysis on an atomic level.
Stainless steel does conduct electricity, though moderately less efficiently than the highest conducting metals like copper or gold. Stainless steel’s alloy mix containing iron, chromium and nickel enables electric current passage. But conductivity performance depends considerably on external and composition factors altering electron mobility.
Metallic Properties of Stainless Steel
Stainless steel is classified as a metal alloy, meaning it combines metallic elemental properties. Its core iron component mixed with chromium and nickel makes stainless steel electrically conductive comparable to iron and steel metals. The alloy’s metallic bonding facilitates electron mobility necessary for current flow.
The inherent malleability, high tensile strength and durability of stainless steel also mirrors that of ductile steel and iron-based metals. These traits enable fabricating an array of stainless items needing capable strength and conductivity like appliances, machinery and architecture.
Inherent Conductivity in Steel
The iron present across all stainless steel grade types represents its source of electrical conductivity. Iron atoms have outer valence electrons that can move by applying external voltage. This allows electrical current transmission through stainless steel comparable to traditional carbon steel.
Stainless steel generally delivers lower conductivity than pure iron or steel, averaging around 2% that of copper’s efficient conduction rates. Varying grade compositions and work hardening stainless during fabrication alters conductivity capacity.
Role of Alloying Elements
Chromium and nickel alloyed with iron in stainless steel can reduce its electrical conduction ability. Elements like chromium contribute additional valence electrons that interact and can impede electrical current flow versus pure iron. But key ratios enable retention of decent conductivity.
Nickel also demonstrates very low conductivity alone. But when added to iron-chromium stainless steel at typical levels under 20%, it avoids severely inhibiting electrons’ ability to move under voltage loads and transmit signals. Overall however, some loss results.
Factors Impacting Conductivity Rate
The conductivity rate of stainless steel gets influenced by mechanical and thermal processing that alters grain structures. Cold working stainless steel hardens the metal but causes electron scattering that lowers conductivity. Annealing heat treatments can restore conductivity but reduce durability.
Environmental factors also affect stainless steel conductivity. Colder temperatures regress conductivity levels. Increased warmth exponentially heightens conduction but scaling amperage loads to avoid failures from resistivity losses proves vital.
Required Voltage Considerations
The volume of electricity stainless steel can conduct depends on the supplied voltage. Adequate voltage must exceed the minimum threshold to motivate electron flow through the inherent resistance stainless possesses as an alloy versus pure conductor metals. It’s crucial to note that stainless steel and aluminum, when in direct contact, may lead to corrosion due to the differing electrochemical potentials.
Insufficient voltage prevents current transmission regardless of stainless steel grade and processing. But exceeding maximum amperage capacity risks irreparable metallic breakdown. Maintaining appropriate conductivity voltage levels for the stainless type and intended currents proves critical.
Effects of Temperature Variations
As temperature rises, stainless steel atoms vibrate more rapidly, enabling easier electron freedom and heightened conductivity. But when extremely cold, atomic motion slows, impeding electron flow. Sudden increases in electrical loads then risk damage from resistance if temperature shifts aren’t controlled.
Cyclic heating and cooling fatigue also degrades stainless conductivity over time through material expansion stresses. Keeping stainless electrical transmission parts at stable room temperatures whenever possible optimizes durable conduction capacity long-term.
Conductivity Across Steel Types
Austenitic stainless steel classes like 304 and 316 containing nickel demonstrate relatively poorer conductivity than ferritic and martensitic stainless variants. Higher chromium also reduces conductivity. But all mainstream stainless types still perform electrical transmission adequately for most general uses.
Precipitation hardening grades can enable heightened conductivity through heat aging treatments facilitating tailored electron mobility. Duplex mixed ferritic-austenitic microstructures also provide improved amperage capabilities from their tightened molecular grain patterns.
Common Conductive Applications
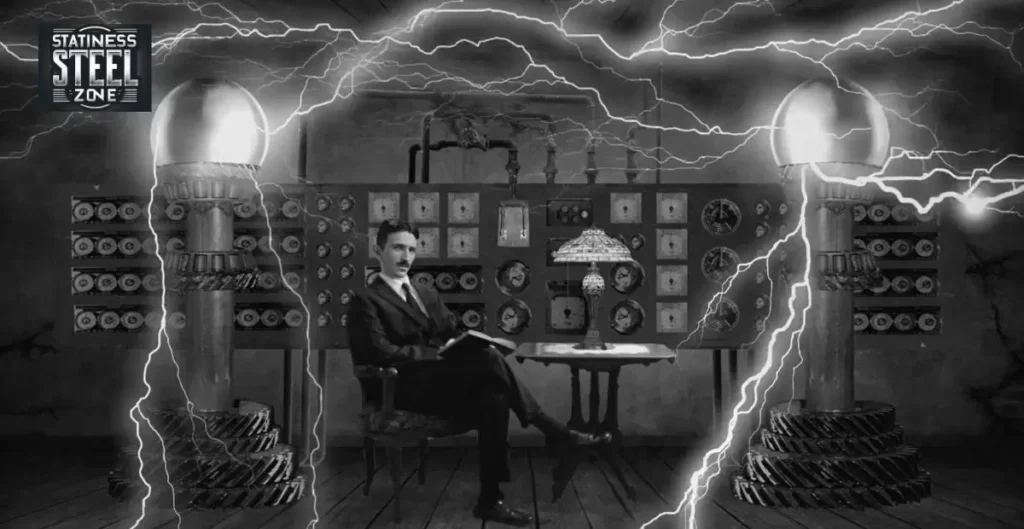
While stainless steel reliably conducts electricity for diverse applications, it’s crucial to note potential corrosion when in contact with aluminum. This interaction is significant in contexts like building facades, aircraft, ships, and appliances, necessitating careful consideration for optimal performance.
Certain conductive capacity limits factor for electrical safety components and distance considerations. But stainless steel effectively replaces traditional carbon steel in numerous conductive applications where weathering resistance proves vital like coastal infrastructure or acidic chemical processing.
Limitations on Amperage & Distance
The maximum electrical current stainless steel can continuously transmit depends on its volume and alloy. But conduction also weakens over sustained distances from accumulating resistive losses. This typically restricts stainless cables to under 65 feet distances needing higher amperage flows.
For safety components like electrical housings or food machinery conductive parts, stainless steel selection warrants precision conductivity specifications matching amperage loads and replacing high performance metals proving environmentally unsuitable.
Improving Conduction Effectiveness
Applying surface lubricative oil coatings including colloidal graphite or molybdenum disulfide improves stainless steel electrical conduction through reduced friction. This enables easier electron flow across contacting stainless components that must transmit signals or power.
Utilizing capacitor-based circuitry or conductive polymers to bridge gaps between stainless steel transmission parts can heighten conduction efficiency. This compensates for alloy-based electron scattering losses that accumulate over distances in cables or throughout structures.
Future Innovations on Conductive Steel
Ongoing metallic alloy development promises tuned stainless steel grades with nickel percentages and treatment processes optimized to heighten electrical conduction versus structural integrity trade offs present in existing alloys.
Adaptive smart conductive coatings that respond to internal voltage loads by geometrically aligning to reduce electron scatter may someday enable stainless steel to approach conductivity levels nearing traditional conductive metals like copper across vast spans.
FAQs
Why does stainless steel show lower conductivity than traditional steel?
Alloying elements like chromium and surface oxidation effects increase stainless steel’s resistivity, impeding higher amperage loads versus plain-iron steel long-term.
How does work hardening during fabrication affect conductivity?
Cold working stainless steel alters grain structures, causing electron scattering that lowers maximum usable amperage capacity compared to annealed variants.
What enables austenitic grades to still conduct electricity?
Despite higher chrome and nickel alloys, austenitic stainless steel retains adequate iron presence and atomic binding to motivate electron mobility when sufficient voltage gets introduced.
Conclusion
Stainless steel’s iron alloy composition enables adequate electrical conductivity for widespread structural and mechanical conduction uses. But processing factors and thermal impacts alter electron flows noticeably. This makes selecting suitable grades and controlling application conditions vital.
Stainless steel’s conduction capacities and limits lets engineers leverage its combined strength and conductivity uniquely. With conductivity maximizing steps, stainless steel performs electricity transmission reliably across the modern structures and devices enabling daily life.